Lithium-ion battery materials are at the heart of today’s technological advancements, powering everything from portable devices to electric vehicles. But what exactly are these materials, and how do they work together to deliver efficient energy storage and release? In this blog, we’ll explore the fundamentals of lithium-ion battery materials, including their key components, manufacturing processes, and performance characteristics. We’ll also delve into the environmental and safety considerations that shape their production and usage. Whether you’re a beginner or an industry professional, join us on this journey to unlock the secrets behind the materials that drive our modern world.
What Are Lithium-Ion Battery Materials? An Introduction for Beginners
Lithium-ion batteries power everything from smartphones to electric vehicles (EVs), but what exactly are they made of? These batteries rely on four key materials to store and release energy efficiently:
Why These Materials Matter
Each component plays a critical role:
- Cathode (e.g., lithium cobalt oxide, NMC, LFP): Determines capacity and voltage.
- Anode (typically graphite or silicon): Stores lithium ions during charging.
- Electrolyte (liquid or solid): Allows ion movement between electrodes.
- Separator: Prevents short circuits while enabling ion flow.
How They Work Together
When charging, lithium ions move from the cathode to the anode via the electrolyte. During discharge, the process reverses, generating electricity.
From Raw Material to Battery Cell: Lithium-Ion Battery Manufacturing Workflow
Step-by-Step Production Process
-
Material Sourcing:
- Lithium, cobalt, nickel, and graphite are mined or recycled.
- Purification ensures high-grade inputs.
-
Electrode Preparation:
- Cathode: Mixed as a slurry (active material + binder + solvent), coated onto foil, and dried.
- Anode: Similar process using graphite/silicon.
-
Cell Assembly:
- Stacked (prismatic/pouch) or wound (cylindrical) with separators.
- Filled with electrolyte and sealed.
-
Formation & Testing:
- Initial charge/discharge cycles activate materials.
- Quality checks for capacity, voltage, and safety.
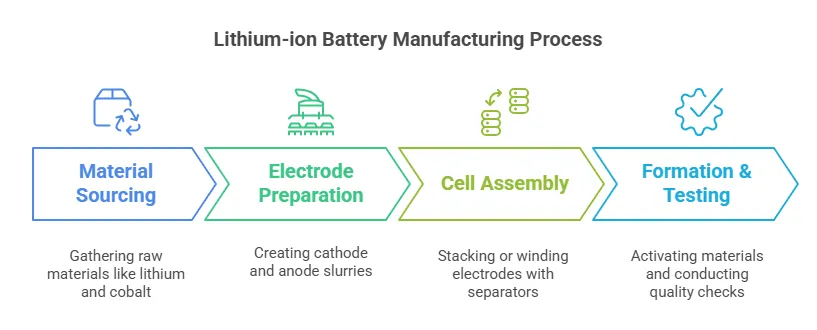
Why Precision Matters
Controlling humidity, temperature, and particle size ensures performance and longevity.
How Lithium-Ion Battery Materials Enable Energy Storage and Release
The Science Behind Ion Movement
- Charging: Lithium ions exit the cathode (e.g., LiCoO₂), traverse the electrolyte, and embed in the anode (graphite).
- Discharging: Ions return to the cathode, releasing electrons to power devices.
Key Material Properties
- High Ionic Conductivity (electrolyte): Faster charging.
- Stable Structure (cathode/anode): Prevents degradation.
- Thermal Resilience: Safety under high loads.
Real-World Impact
- EVs use nickel-rich cathodes for range.
- LFP (lithium iron phosphate) prioritizes safety for grid storage.
High-Nickel vs. LFP Materials: Choosing the Right Chemistry for Your Use Case
What Defines Each Chemistry?
-
High-Nickel (NMC/NCA):
- Pros: Higher energy density (longer EV range).
- Cons: Costly, thermal instability risks.
-
LFP (Lithium Iron Phosphate):
- Pros: Cheaper, longer lifespan, safer.
- Cons: Lower energy density (heavier batteries).
How to Decide?
Use Case | Recommended Chemistry |
---|---|
Electric Vehicles | High-nickel for range |
Home Energy Storage | LFP for safety & cost |
Consumer Electronics | Balanced NMC |
Why Trends Shift
Tesla’s adoption of LFP for standard-range EVs highlights cost vs. performance trade-offs.
Environmental Considerations and Safety Protocols in Battery Material Processing
Why Environmental Impact Matters
Lithium-ion battery production involves mining, chemical processing, and waste generation, raising concerns about:
- Resource depletion (e.g., lithium, cobalt).
- Water contamination from solvent use.
- Carbon emissions from high-temperature processing.
How Manufacturers Reduce Harm
- Recycling Programs: Recovering lithium/nickel from spent batteries.
- Dry Electrode Coating: Eliminates toxic solvents (used by Tesla).
- Renewable Energy: Powering facilities with solar/wind to cut emissions.
Step-by-Step Safety in Material Handling
- Hazard Identification: Classify chemicals (e.g., HF acid in cathode production).
- Engineering Controls: Use sealed reactors and ventilation.
- PPE Requirements: Respirators, gloves, and flame-resistant suits.
- Waste Disposal: Neutralize acids before landfill.
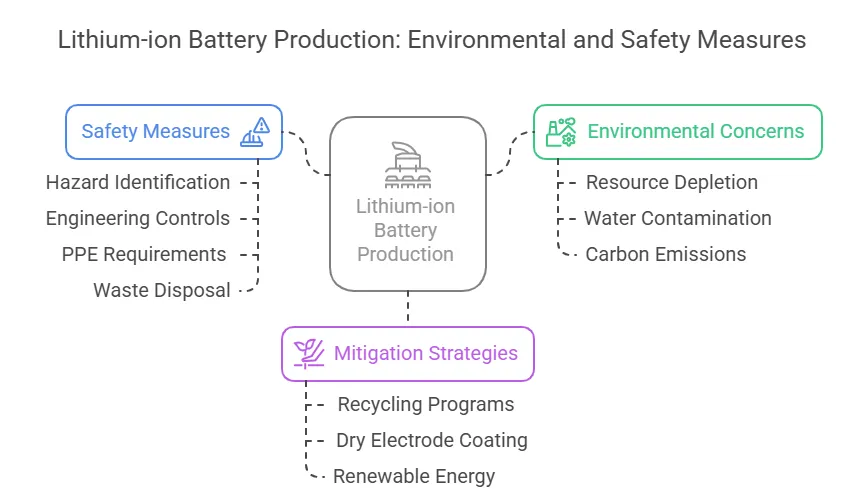
How Lithium-Ion Battery Materials Are Tested for Purity and Performance
What Tests Are Conducted?
-
Purity Analysis:
- ICP-MS (Inductively Coupled Plasma Mass Spectrometry) detects trace metals.
- XRD (X-ray Diffraction) checks crystal structure defects.
-
Performance Testing:
- Cycle life (charge/discharge under controlled temps).
- Rate capability (fast-charging simulation).
Why Testing Is Critical
- Impurities (e.g., iron in graphite) cause dendrites, risking shorts.
- Inconsistent particle size reduces energy density.
How Labs Validate Materials
- Sample Preparation: Crush and homogenize cathode powder.
- Accelerated Aging: Expose to 60°C/80% humidity for 2 weeks.
- Post-Test Analysis: Measure capacity loss via electrochemical impedance spectroscopy.
Material Safety Data and Precautions for Lithium-Ion Battery Components
What Hazards Exist?
Component | Risks | Precautions |
---|---|---|
Lithium Cobalt Oxide | Toxic if inhaled; carcinogenic. | Use fume hoods, NIOSH masks. |
Organic Electrolyte | Flammable; reacts with water. | Store in argon-filled dry rooms. |
Graphite Dust | Explosive in air. | Anti-static flooring, spark-proof tools. |
How to Handle Spills
- Electrolyte Leaks: Absorb with vermiculite, avoid water.
- Metal Powders: Smother with Class D fire extinguishers (never CO₂).
Why Training Is Non-Negotiable
- OSHA mandates Hazard Communication Standard (HCS) training for workers handling LiPF₆ electrolyte.
Best Practices for Maintaining Lithium-Ion Battery Material Storage Facilities
What Ideal Storage Looks Like
- Temperature: 15–25°C (prevent electrolyte degradation).
- Humidity: <30% RH (moisture ruins anode slurry).
- Ventilation: Continuous airflow to disperse fumes.
How to Organize Materials
-
Segregation:
- Keep cathodes (oxidizers) away from anodes (flammable).
- Store lithium metal separately under mineral oil.
-
Inventory Control:
- FIFO (First-In-First-Out) to avoid expired materials.
- RFID tags for real-time tracking.
Why Compliance Audits Matter
- NFPA 855 (fire codes) and IATA regulations (for shipping) require:
- Firewalls between storage units.
- Thermal runaway containment systems.
This in-depth exploration of lithium-ion battery materials covers everything from their basic components—cathodes, anodes, electrolytes, and separators—to the complex manufacturing processes that transform raw materials into functional battery cells. We examine the science behind ion movement and the critical properties that enable high-performance energy storage and release. The blog also compares different chemistries, such as high-nickel and LFP materials, highlighting their unique advantages and trade-offs for various applications. Additionally, we address the environmental impact of battery production and the safety protocols necessary for handling these materials. Finally, we outline best practices for material storage and maintenance to ensure optimal performance and longevity. This guide is essential reading for anyone interested in understanding the core elements and future potential of lithium-ion battery materials.