Reasons for the Capacity Degradation of Lithium Batteries
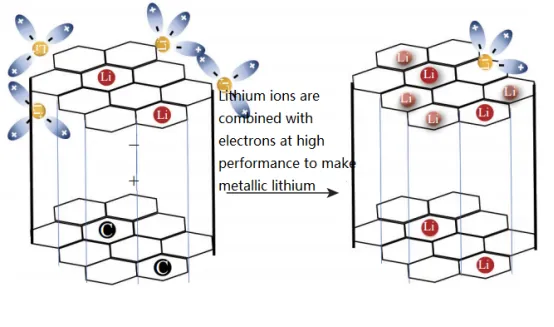

Follow me on:
1.lithium precipitation and SEI film in lithium batteries
This article provides a comprehensive analysis of the capacity degradation mechanism in lithium-ion batteries. It categorizes and organizes the factors that influence the aging and lifespan of lithium-ion batteries. It elaborates in detail on various mechanisms such as overcharging, SEI film growth and electrolyte, self-discharge, active material loss, and current collector corrosion. The article summarizes the research progress in battery aging mechanisms by scholars from various fields in recent years. It thoroughly analyzes the influencing factors and modes of action in lithium-ion battery aging and discusses modeling methods for aging side reactions.
1.1 Classification and Impact of Aging Factors in Lithium-ion Batteries
1.1.1 Classification of Aging Factors in Lithium-ion Batteries
The aging process of lithium-ion batteries is influenced by various factors, including their configuration in electric vehicles, environmental temperature, charge/discharge rates, and depth of discharge. Capacity and performance degradation are typically the result of multiple side reactions and are associated with numerous physical and chemical mechanisms. The mechanisms of degradation and forms of aging are highly complex.
1.1.2 The aging effects on lithium-ion batteries
The main external manifestations of lithium-ion battery aging are decreased usable capacity and increased internal resistance. This leads to a decline in the actual charge/discharge capacity and the maximum available power of the battery.
Furthermore, the increase in internal resistance results in issues such as increased heat generation, elevated module temperatures, and increased temperature inconsistency during usage. This necessitates improved thermal management systems for lithium-ion batteries. Additionally, the side reactions occurring within the lithium-ion battery can vary due to factors such as battery configuration and connection structures, leading to differences in operating conditions among individual cells. As the battery is used, the aging rates of the cells can differ, exacerbating the inconsistency issues in lithium-ion battery packs.
The open-circuit voltage (OCV) curve of a lithium-ion battery represents the internal electromotive force of the battery at a given state. As a lithium-ion battery ages, the OCV curve may experience a certain degree of shift or deformation compared to its original state. This leads to changes in the actual charge/discharge voltage curve of the battery, affecting the accuracy of battery state estimation in practical usage scenarios. With aging, the maximum charge/discharge rate of the lithium-ion battery also decreases. If the battery management system does not adaptively adjust, there is a risk of overcharging, over-discharging, and exceeding power limits, increasing the safety risks associated with lithium-ion battery usage.
1.2 Lithium-ion battery capacity decline mechanism
1.2.1 Analysis of the impact of capacity decline caused by lithium precipitation
Figure 1 illustrates the loss of active lithium ions caused by lithium precipitation at the negative electrode. lithium precipitation refers to the process in which lithium from the electrolyte deposits onto the electrode surface. The occurrence of lithium precipitation on the negative electrode surface is a significant aging factor in lithium-ion batteries and also a crucial factor affecting battery safety. When the negative electrode potential exceeds a threshold of 0V (relative to Li/Li+), lithium precipitation occurs on the surface of the negative electrode.
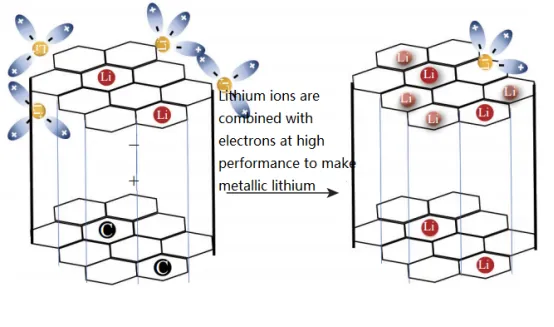
lithium precipitation leads to irreversible loss of lithium ions, resulting in a decrease in available capacity. The diagram in Figure 2 illustrates the loss of active lithium ions caused by the growth of lithium precipitation. Some researchers suggest that both a slow rate of lithium ion insertion into the graphite negative electrode and a fast rate of lithium ion transport to the negative electrode can trigger lithium precipitation. Furthermore, studies have shown that working at low temperatures can result in a slower diffusion rate of lithium ions, and when the working potential of the negative electrode is very close to the dendrite formation potential, it is more likely to lead lithium precipitation. Additionally, having a low N/P ratio (the ratio of negative electrode capacity to positive electrode capacity) can lead to lithium precipitation, as well as localized electrode polarization and geometric mismatch.
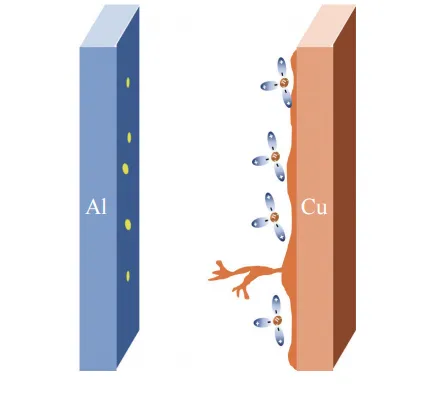
2.The growth of the SEI film and its impact on capacity degradation
The SEI film is a passivation layer that forms on the surface of the negative electrode in a lithium-ion battery. It exhibits ion conductivity while blocking electron flow, effectively separating the electrolyte from the negative electrode. The growth of the SEI film is a major side reaction at the interface between the negative electrode and the electrolyte in lithium-ion batteries. It leads to irreversible capacity loss and significantly affects the battery’s rate capability, lifespan, and safety characteristics. Under normal operating conditions, the growth of the SEI film is the primary factor causing the loss of active lithium in the battery.
The formation of the SEI film is also one of the main causes of calendar aging, especially at higher temperatures and higher state of charge (SOC). Compared to the SEI film formed in new batteries and under normal temperature cycling, the SEI film formed at higher temperatures exhibits better thermal stability and higher density. This can effectively slow down the aging process of the battery. While the growth of the SEI film on the negative electrode may have negative effects on the capacity and internal resistance of lithium-ion batteries, a stable SEI film can improve the electrode material interface properties and contribute to better cycling performance of the battery. Some researchers also propose a dual-layer structure of SEI film, consisting of a dense inner layer (initial SEI film) and a porous outer layer (long-term growth layer), to better explain the impact of the SEI film on battery characteristics.
3.Corrosion of current collectors
3.1 Capacity loss due to corrosion of current collectors
Corrosion of the current collector can lead to a reduction in battery lifespan and impact its stability and safety. Under extreme conditions such as over-discharge, where the voltage drops to around 1.5V, copper in the electrolyte can be oxidized, resulting in the dissolution of copper from the current collector. The oxidized copper ions can then deposit as metallic copper during subsequent charging cycles, leading to the formation of a thick copper layer on the surface of the negative electrode material. This copper deposition hinders the intercalation and deintercalation of lithium in the negative electrode and contributes to the thickening of the SEI (Solid Electrolyte Interphase) film, ultimately causing capacity decay in the lithium-ion battery.
4.Decomposition of electrolyte and separator
4.1 The effect of electrolyte decomposition on capacity degradation
The electrolyte in lithium-ion batteries serves as an ion conductor, facilitating the movement of lithium ions between the positive and negative electrodes. However, as the number of charge and discharge cycles increases, the electrolyte undergoes oxidation or decomposition reactions over time. This leads to a reduction in its ion conductivity, resulting in increased internal resistance within the battery.
In addition to reacting with the surfaces of the positive and negative electrodes of the battery, the electrolyte will also undergo a series of reactions during lithium precipitation and heating. When heated, the electrolyte may decompose and generate gases such as CO2. Further increases in temperature may even lead to combustion and explosion.
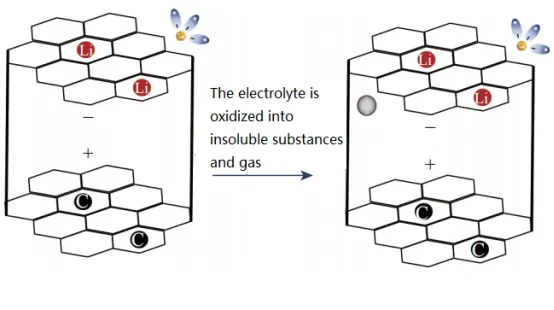
5.Analysis of the Impact of Temperature, Charge/Discharge Rate, and Overcharging on Capacity Degradation
5.1 Temperature
When working at higher temperatures, due to reaction kinetics (Arrhenius effect), the electrochemical reaction rate of lithium-ion batteries increases, the internal resistance decreases, and the capacity increases; sustained higher temperatures will cause the internal side effects of the battery to increase. The reaction accelerates, causing oxidation and decomposition of the electrolyte and promoting the formation of SEI film, causing irreversible loss of capacity and increase in impedance. During the operation of the lithium-ion battery, due to the low thermal conductivity of its internal electrodes, separators and other components, a temperature gradient will occur inside the battery cell. The temperature gradient phenomenon is more obvious at high magnification and low temperature environments. This difference in spatial temperature distribution This property may aggravate the non-uniform distribution of current density, thereby accelerating battery degradation.
5.2 Charge/Discharge rate
Current rate will also cause the capacity of lithium-ion batteries to decrease. The increase in charge and discharge rate will accelerate the capacity fading rate of high specific energy lithium-ion batteries and the growth rate of ohmic internal resistance and polarization internal resistance. The growth rate of polarization internal resistance is higher than the ohmic internal resistance.
5.3 Analysis of the impact of overcharging on capacity degradation
When the negative electrode is overcharged, lithium precipitation occurs, leading to the deposition of metallic lithium. This phenomenon is more likely to occur when there is an excess of active material in the positive electrode compared to the negative electrode. However, even in cases where the ratio of active materials between the positive and negative electrodes is normal, lithium precipitation can still occur during high-rate charging. The deposition of metallic lithium can cause capacity degradation in the following ways: ①Reduction in Available Lithium; ②The precipitated metallic lithium reacts with the solvent or electrolyte to form other by-products and consumes the electrolyte, thereby reducing the discharge efficiency; ③The metallic lithium is mainly deposited between the negative electrode and the separator, which may cause the pores of the separator to be clogged, causing the battery to resistance increases.
When the proportion of active materials in the positive electrode is too low compared to the negative electrode, overcharging of the positive electrode is prone to occur. Overcharging of the positive electrode mainly leads to capacity degradation of the battery through the generation of electrochemically inert substances and oxygen loss. As it disrupts the capacity balance between the electrodes, irreversible capacity loss can occur in the battery. Additionally, the release of oxygen from the positive electrode reaction can pose safety risks to the use of lithium-ion batteries.